
EV Battery Chemistry: NCM, NCA, LFP, Solid State
Key Insights:
NMC, NCA, LFP, and emerging solid-state batteries are crucial in EV battery chemistry, each with unique pros and cons.
NMC batteries offer high energy density and versatility but raise concerns about cobalt scarcity and safety.
NCA batteries boast high energy density but face challenges with cobalt usage and temperature sensitivity.
LFP batteries prioritize stability and safety, ideal for applications prioritizing durability over raw energy density.
Future technologies like lithium-air and solid-state batteries show potential for significantly higher energy capabilities, promising to revolutionize the automotive industry.
The widespread adoption of electric vehicles in India is driven by their environmental sustainability and fuel efficiency. However, the technological limitations of high-voltage batteries hinder the progression to full EV dominance in transportation. Despite significant advancements in battery technology over the past decade, current batteries still lack the energy density of liquid fuels. Pursuing a battery with substantially higher energy density remains a paramount goal in automotive research. This review explores existing and upcoming battery technologies, shedding light on their functionalities and potential impact on the EV landscape.
Planning to set up a Charging station?
Connect with 1C for expert advice on EV chargers
What is an EV Battery?
An EV battery, or electric vehicle battery, is a rechargeable energy storage device used to power electric vehicles. These batteries store electrical energy and supply it to the vehicle’s electric motor, enabling it to drive. EV batteries are typically composed of multiple cells connected in series. They are designed to provide the necessary energy to propel the vehicle while offering sufficient range for various driving needs.
Types of EV Battery
EV Batteries are divided into various categories:
- Lead Acid Battery ( Wet Cell Battery)
- Deep Cycle Battery
- SLI Battery
- VRLA Battery
- Silver Calcium Battery
- Enhanced Flooded Battery (EFB)
- Lithium-Ion Battery
- Nickel Metal Hydride Battery (NiMH Battery)
- Sodium-Ion Battery
We have discussed about each type in detail in our blog, Types of Car Battery.
What is EV Battery Chemistry?
An EV battery chemistry refers to the composition and materials used in the construction of batteries for electric vehicles. It encompasses various types of battery technologies, such as lithium-ion NCM, NCA, and LFP cathodes, as well as potential future advancements like lithium-air and solid-state batteries. These chemistries determine factors like energy density, longevity, and performance of the batteries, influencing their suitability for different applications in electric vehicles. Let us discuss each EV Battery Chemistry one by one in detail.
Lithium-Ion NMC
Lithium-ion NMC cathode batteries represent a significant advancement in battery technology, widely used in electric vehicles due to their favourable characteristics. Comprising nickel, manganese, and cobalt, NMC batteries offer enhanced performance and efficiency compared to traditional alternatives.
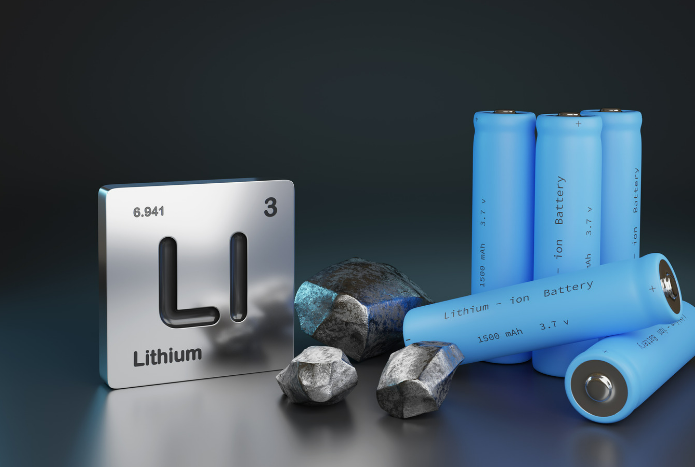
The functionality of Lithium-Ion NMC Cathode Batteries
NMC batteries function by facilitating the movement of lithium ions between the anode and cathode during charging and discharging cycles. This process involves the transfer of lithium ions from the anode to the cathode during discharge while electrons travel through the external circuit. Upon charging, the opposite occurs, with lithium ions moving from the cathode to the anode.
Advantages and Disadvantages of NMC Battery
Advantages | Disadvantages |
Higher energy density | Allows for more energy storage in a compact space. |
Versatile composition for tailored performance | Enables customisation based on specific application needs. |
Long lifespan | Offers a prolonged operational life, reducing replacement frequency. |
Environmental benefits | Positively contributes to environmental sustainability. |
Reduced reliance on fossil fuels | Increased production costs may impact affordability. |
Resource constraints, especially with materials like cobalt. | |
Safety concerns related to thermal runaway events. |
Lithium-Ion NCA
Lithium-ion NCA (Nickel-Cobalt-Aluminum) batteries significantly advance electric vehicle battery technology. These batteries, characterised by their cathode composition, have played a pivotal role in the proliferation of EVs globally.
Composition
- Nickel (N): NCA cathodes contain a significant proportion of nickel, enhancing the battery’s overall energy density. Higher nickel content is associated with improved efficiency and reduced battery pack weight.
- Cobalt (C): Despite concerns about the ethical and environmental implications of cobalt mining, NCA cathodes traditionally include cobalt. However, efforts are underway to minimise cobalt usage or exclude it from the cathode composition.
- Aluminum (A): Aluminum is another essential component in NCA cathodes. It contributes to the cathode’s stability and helps maintain the structural integrity of the battery during charge and discharge cycles.
Characteristics
- High Energy Density: Lithium-Ion NCA batteries offer impressive energy density, crucial for extending electric vehicle range.
- Longevity: NCA cathodes demonstrate good cycling stability, ensuring a reasonable number of charge cycles before degradation.
- Temperature Sensitivity: NCA batteries, like others, are sensitive to temperature changes, requiring proper thermal management to prevent overheating.
Advancements and Challenges
- Reducing Cobalt Content: Ongoing research focuses on minimising or eliminating cobalt from NCA cathodes due to concerns about its scarcity and ethical mining practices. This shift aims to create more sustainable and environmentally friendly battery technologies.
- Increased Nickel Content: Manufacturers are exploring higher nickel content in NCA cathodes to enhance energy density further and improve the overall efficiency of electric vehicle batteries.
Lithium-Ion LFP
LFP batteries use lithium iron phosphate as the cathode material. This variant has gained attention from manufacturers for specific reasons despite having performance levels generally lower than other lithium-ion types.
Composition and Chemistry
The cathode material, lithium iron phosphate (LiFePO4), is known for its stability and safety. Iron is abundant and less prone to ethical concerns, unlike other cathode materials like cobalt, making LFP an environmentally preferable option.
Performance Characteristics
While the specific energy density of LFP batteries is generally lower than other lithium-ion types, there are key advantages. LFP batteries exhibit a longer service life, making them an appealing choice for applications prioritising durability over raw energy density.
Factors Influencing Performance:
- Voltage Limitations: LFP batteries have a lower maximum voltage, resulting in a reduced kilowatt-hour capacity for the same physical size compared to other lithium-ion types.
- Spatial Dimension of Ion Movement: Lithium ions in LFP batteries move in one spatial dimension, unlike the two-dimensional movement in NMC batteries. This limits the maximum discharge power, making LFP batteries less suitable for high-performance applications.
Application by Tesla
Tesla, a prominent electric vehicle manufacturer, incorporates LFP batteries in the base version of its Model 3. While LFP batteries may not be optimal for high-performance scenarios, they find utility in certain vehicle configurations.
Charging Recommendations
One distinctive feature of LFP batteries is their tolerance for charging to 100%. Unlike some other lithium-ion types, charging LFP batteries to full capacity is not only acceptable but is often recommended for optimal performance and longevity.
Planning to set up a Charging station?
Connect with 1C for expert advice on EV chargers
Lithium-Air and Solid State Batteries
As the world continues its transition towards sustainable energy solutions, the future of transportation hinges on advancements in battery technology. The quest for more efficient, longer-lasting, and environmentally friendly batteries has led to ongoing research and development in the field. In this rapidly evolving landscape, two potential game-changers stand out: lithium-air and solid-state batteries.
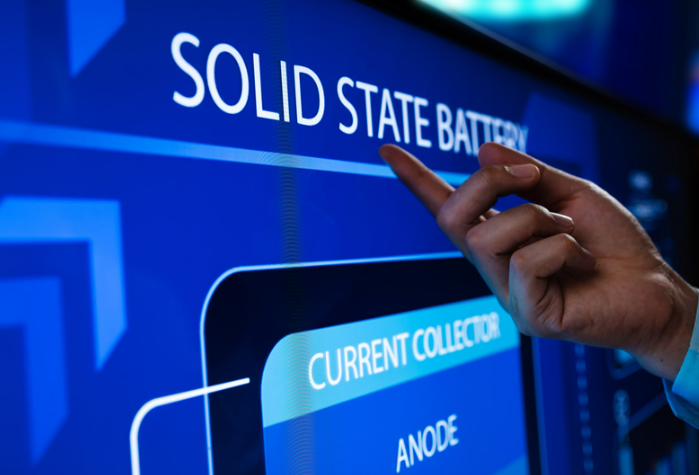
Lithium-Air Batteries
With a potential energy capability rivalling liquid fuels (11.4 kWh/kg), lithium-air batteries could transform the automotive industry. They use lithium in the anode, air in the cathode, and an electrolyte. Challenges like high charging temperatures and dendrite formation limit current applications to labs. Overcoming these challenges could render internal combustion vehicles obsolete.
Solid State Batteries
Solid-state batteries replace liquid electrolytes with solid ones. While not in current electric cars, projections anticipate their introduction within two to three years. Expected benefits include double the energy density of conventional lithium-ion batteries and a lifespan of up to 1000 cycles, subject to refinement through ongoing research.
Conclusion
The advancement of EV battery technology is crucial for the widespread adoption of EVs. Various battery chemistries, such as lithium-ion NMC, NCA, LFP, and emerging solid-state batteries, offer different advantages and challenges. Each chemistry contributes to the overall goal of improving EV performance, range, and sustainability. Continued research and development in battery technology are essential for driving the EV industry forward and achieving a greener transportation future.
FAQs
Which battery chemistry is best for EV?
There isn’t a single “best” battery chemistry for electric vehicles, as each chemistry has its own set of advantages and limitations. Some commonly used battery chemistries for EVs include lithium-ion (Li-ion) NMC (nickel-manganese-cobalt), lithium-ion LFP (lithium iron phosphate), and solid-state batteries. The choice depends on factors like energy density, cost, safety, and environmental impact.
What is the main ingredient in EV batteries?
The main ingredient in most EV batteries is lithium. Lithium is used in various forms, such as lithium-ion, lithium iron phosphate, and other lithium-based chemistries. It serves as the primary material for the battery’s anode, facilitating the movement of ions during the charging and discharging processes.
What are the new EV battery chemistries?
New EV battery chemistries like lithium-sulfur, lithium-air, and solid-state batteries are under development to boost performance and energy density. They aim to overcome current limitations, offering better range, faster charging, and longer lifespan. However, extensive testing and refinement are needed before widespread use.